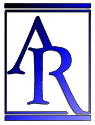 |
Annu. Rev. Astron. Astrophys. 1992. 30:
705-742
Copyright © 1992 by Annual Reviews. All
rights reserved |
4. ACQUISITIONS & ACCRETIONS
Galaxies can acquire material from passing companions via mass
transfer or accrete much less-massive neighbors, such as satellites,
suffering only relatively minor damage. Although individual events
like these add only a modest amount of mass to large galaxies, their
consequences can have a significant effect on observable properties of
galaxies and provide indications as to the frequency and import of
galaxy collisions.
Shells & Other Fine Structures
Beginning with Arp's (1966) classic study of peculiar galaxies, ``fine
structures,'' such as ``shells,'' ``ripples,'', ``plumes,'' boxy
isophotes, and ``X-features'' have been detected in an ever-larger
fraction of S0's and ellipticals
(Malin & Carter 1980,
1983;
Schweizer 1980). Indeed, in the
most recent survey by
Seitzer & Schweizer (1990), 32%
of their S0's and 56% of their E's
possess shells and ~ 10% and ~ 30% of their entire sample
have X's and boxy isophotes, respectively. The actual percentage of
all galaxies exhibiting such fine structure is rather uncertain, owing
to the difficulty of determining if the faint features detected are
bona fide. This complication is especially acute for later-type
disks, which often possess diffuse spiral patterns that mimic,
e.g. shells. However, there are observational indications that
shells exist in at least some Sa and Sb galaxies (e.g.
Schweizer & Seitzer 1988).
Simulations employing restricted methods support the view that the
accretion of material by large galaxies provides a natural explanation
for the origin of fine structures. Shells can form either by the
``phase-wrapping'' of debris on nearly radial orbits
(Quinn 1984) or
by the ``spatial-wrapping'' of matter in thin disks
(Hernquist & Quinn
1988). Contrary to
Quinn's (1984) hypothesis, the
shell-forming
material need neither reside initially in a thin disk nor be on
precisely radial orbits. Shells can be produced during the accretion
of spheroidal companions by larger galaxies, provided that the total
phase-space volumes they occupy are sufficiently different
(Dupraz & Combes 1986;
Hernquist & Quinn 1988), and in
non-radial collisions
through mass transfer, eliminating concerns that an improbable
encounter geometry would be needed to produce them
(Toomre 1983;
Hernquist & Quinn 1988).
These various studies have also shown that other types of fine
structure develop in encounters which simultaneously produce shells.
Plumes consisting of debris on unbound or weakly-bound orbits occur
frequently in shell-forming collisions
(Hernquist & Quinn 1988).
Moreover, material accreted by non-spherical potentials can produce
X-structures and features that appear boxy in projection
(Hernquist & Quinn 1989).
In spite of the general acceptance of the accretion model, a number of
difficulties remain. The numerical studies mostly ignore self-gravity
and the consequences of dynamical friction. While the self-gravity
of the victim does not appear crucial to the issue of whether or not
fine structures will form
(Barnes 1989;
Heisler & White 1990;
Salmon et al. 1990), it
is impossible to predict, e.g. the radial
distribution of shells formed by a close collision without including
dynamical friction
(Dupraz & Combes 1987). Indeed,
if the accretion
scenario is correct, it appears that dynamical friction probably
played a significant role in structuring some of these objects since
many contain extremely tightly bound shells. Self-consistent
calculations by
Heisler & White (1990) indicate
that the disruption
of satellites can indeed inject material onto tightly bound orbits,
populating inner shells. However, even these models are not
sufficiently detailed to explain objects such as
NGC 3923, which
contains more than 20 shells distributed regularly around the galaxy.
As noted by Thomson & Wright
(1990), the restricted simulations are
deficient in other regards. In some galaxies, the intensity of shells
increasingly far from the galaxy drops similarly to that of the
elliptical, suggesting that these shells may be of internal
origin. Accordingly, Thomson & Wright have put forward an alternate
model in which shells are induced in a thin disk component of an
elliptical by a tidal encounter with another galaxy. The existence of
such disks in ellipticals is problematic, but the inability of
restricted calculations like those of
Hernquist & Quinn (1988) to
explain the radial luminosity of shell systems re-emphasizes the need
for more physical models.
It should also be noted that boxy or X-shaped structures need not
arise through accretion events; these features simply reflect
phase-mixed populations of stars in certain kinds of orbits which may
have been created by a variety of processes
(Binney & Petrou 1985).
An example is provided by the recent work of
Merritt & Hernquist (1991) who
show that dynamical ``bending'' instabilities produce
remnants which are photometrically similar to ellipticals having boxy
isophotes and weak X-structures. These models do not display shells,
however, so a strong correlation between all these various features
would support the view that they are of external origin.
Polar Rings
Many S0 galaxies possess rings of gas-rich material that appear to be
kinematically distinct from the galaxy proper (e.g.
Schweizer et al.
1983;
Athanassoula & Bosma 1985). The
recent survey by
Whitmore et al. (1990)
implies that ~ 0.5% of all S0's have polar
rings; i.e., rings of gas, dust, and young stars with axes
aligned perpendicular to the major axis of the disk. The actual
fraction of S0's with such features may be even larger when
observational selection effects and ring lifetimes are taken into
account.
Searches for similar features in elliptical galaxies have met with
mixed success. Obscuring dust lanes (e.g.
Bertola 1987) and diffuse,
extended disks and rings of gas are seen in many ellipticals (e.g.
van Gorkom et al. 1986;
Lake et al. 1987;
Kim et al. 1988;
Schweizer et al. 1989),
but few ellipticals with rings as substantial as those
of classical polar rings in S0's are known. A notable exception is the
galaxy AM2020-5050
(Whitmore et al. 1987),
which is classified as
Hubble type E4. However, this object rotates rapidly and appears
somewhat intermediate between an elliptical and a S0 galaxy.
Since these rings often appear kinematically distinct from their
hosts, it is generally assumed that they are of external origin. Two
scenarios have been proposed for the formation of polar rings:
accretion of gas-rich dwarfs (e.g.
Athanassoula & Bosma 1985) and
capture of material from a passing galaxy by mass transfer. An
example of the latter is
NGC 3808, where a stream of material can
be seen linking a large disk galaxy to an S0 of comparable luminosity
as shown in Figure 1. Unfortunately,
neither scenario has been
tested in detail. Theoretical studies of polar rings have instead
focussed mainly on determining the equilibrium states accessible to
material on closed orbits in axisymmetric and triaxial potentials.
These calculations have shown that the ``settling time,'' which is
the time for gas in an axisymmetric or triaxial potential to settle
into steady state, is small compared to the age of the universe
(Tohline et al. 1982;
Steiman-Cameron & Durisen 1982,
1988;
Habe & Ikeuchi 1985,
1988;
Varnas et al. 1987), and
so there is sufficient
time for accreted gas to form polar rings.
However, all these studies are limited by their neglect of
self-gravity and time-dependent effects and their use of unrealistic
initial conditions. The first two difficulties will likely be
overcome by Lagrangian codes, such as TREESPH
(Hernquist & Katz 1989)
which explicitly include gravitational forces between mass elements.
Some preliminary steps in this direction have already been taken by
Rix & Katz (1991). The role of
initial conditions in ring-forming
events is not likely to be elucidated without resort to
calculations which treat the dynamics of galaxy collisions and mergers
in detail, including dynamical friction and dissipation. Such models
may eventually determine why rings are most common in S0 galaxies,
largely avoiding other galactic types, and also explain why fine
structures such as shells should be more common than rings if all
result from accretion of external matter.
Sinking Satellites
As emphasized by
Ostriker & Tremaine (1975)
and Tremaine (1981),
dynamical friction will cause the orbits of satellites around massive
galaxies to decay. Eventually, the victims will be destroyed tidally
and/or cannibalized by the primary galaxy. Using Chandrasekhar's
treatment of dynamical friction, Tremaine showed that galaxies like
the Milky Way have probably accreted a non-negligible amount of mass
in the form of discrete objects over a Hubble time. Although it is
difficult to make precise estimates of this effect, owing to the
absence of a complete theory of galaxy formation and large-scale
structure, Tremaine's simple argument implies that galaxies do not age
peacefully in isolation, even if they do not experience a strong
encounter with a comparably-massed neighbor.
In the limit of weak encounters, perturbation theory can be used to
compute torques on satellites and predict their decay paths
(Tremaine & Weinberg 1984;
Weinberg 1986;
Statler 1988). More generally, there
is little alternative to numerical simulation, particularly if the
details of the tidal disruption of the companion and/or the
self-consistent response of the primary to large perturbations are of
interest. Numerical studies have included the orbital decay of
satellites around spherical galaxies
(Lin & Tremaine 1983;
White 1983a) and disks
(Quinn & Goodman 1986;
Quinn et al. 1991). The
results are supported by quasi-analytic calculations, implying that
simulations yield reliable decay rates
(Hernquist & Weinberg 1989).
However, existing studies have typically approximated the
self-consistent response of the primary, complicating their
interpretation. For example, while
Quinn et al. (1991)
include the self-gravity of primary disks, they ignore the self-consistent
response of the halo. As emphasized by
Barnes (1988), orbital decay
in composite systems involves a complex interplay amongst the various
components. Fully self-consistent calculations by Hernquist (1992)
support this claim and show that the torque on a satellite near a disk
is derived in roughly equal measure from the disk and halo; provided,
of course, that galaxies possess dark matter halos similar to those
inferred in external spirals.
Technical issues aside, it seems likely that a variety of apparently
disparate phenomena may be blamed on satellite accretions. Some
ellipticals contain cores which are kinematically distinct from the
surrounding galaxies (e.g.
Efstathiou et al. 1982;
Franx & Illingworth 1988;
Franx et al. 1989;
Jedrzejewski & Schechter 1988;
Wagner et al. 1988;
Bender 1990a). One possible
explanation for these
peculiar velocity fields was first proposed by
Kormendy (1984) who
argued that the central rotation of
NGC 5813 is a consequence of the
accretion of a spinning dwarf galaxy. This idea was tested by
Balcells & Quinn (1990) using
N-body simulations. In their models,
angular momentum is transferred from the orbit of the dwarf to the
primary by gravitational torques during a merger. If the satellite's
orbit is retrograde, the angular momentum deposited can partly cancel
the primary's original rotation and even reverse it near the center
where the rotation curve is rising. Following mergers from retrograde
orbits, therefore, the central regions of the primary can display
distinct velocity fields from the outlying regions. Although this
specific scenario is not the only way to produce kinematically
decoupled cores, the general idea that angular momentum deposited by
satellite accretions can ``organize'' the shapes and velocity fields
of elliptical galaxies seems promising (e.g.
Quinn et al. 1990).
Satellite mergers may also alter structure of disk galaxies (for a
review, see Hernquist 1991a).
The self-consistent models of
Quinn et al. (1991)
indicate that decaying satellites can excite transient
warps in the outer parts of disks that may be sufficiently long-lived
to explain some stellar warps in external spirals. Moreover,
dynamical heating by these satellite accretions produce disturbed,
featureless disks possessing little or no large-scale spiral
structure
(Hernquist 1989a,
b), reminiscent of amorphous galaxies
(Sandage & Brucato 1979).
Tidal debris from cannibalized satellites
may account for a number of peculiar features in our Galaxy, including
its two-component system of globular clusters (e.g.
Freeman 1990),
retrograde halo stars, and the Magellanic Stream (e.g.
Lin & Lynden-Bell 1982).
More generally, the consequences of satellite accretions are important
for theories of the formation of galaxies and large-scale structure.
Schweizer (1990) has proposed that the bulges of spiral galaxies may
be relics of past accretion events. A similar suggestion has been
made for thick disk components of external, edge-on systems (for a
discussion, see
Quinn et al. 1991). Such
processes may be responsible
for many aspects of galactic structure that were once attributed to
events at the time galaxies were born. If so, then it is impossible
to relate the observed properties of galaxies to cosmological models
without considering late and on-going evolutionary effects. Indeed,
as noted recently by
Toth & Ostriker (1992) the
existence of cold,
thin disk components in spiral galaxies limits the rate of satellite
accretion in cosmological models. Whether or not these constraints
can be applied to test actual scenarios for the formation of
large-scale structures remains to be seen.